Carbon Dynamics in New Zealand’s Native Forests
Native forests play an important role in storing and sequestering carbon in the New Zealand landscape. They do not sequester carbon as quickly as pine forests but can provide significant carbon stores and sinks, as well as a range of other benefits.
The relationship between plants and carbon
All living things, including plants, contain carbon. Via photosynthesis, a plant takes carbon dioxide (CO2) from the atmosphere, and converts the carbon (C) into sugars which are stored in the trunk, stems, branches and foliage (i.e. within the biomass of the plant). The carbon stored is released back into the atmosphere when the plant dies and decays or is burned.
The amount of carbon stored in a plant is proportional to its size and density. Smaller, herbaceous (non-woody) plants contain relatively little carbon while large trees, with woody trunks and stems, contain the most. Around 50% of the dry mass [1] of a tree is carbon. The faster a tree grows, the faster it removes carbon from the atmosphere.
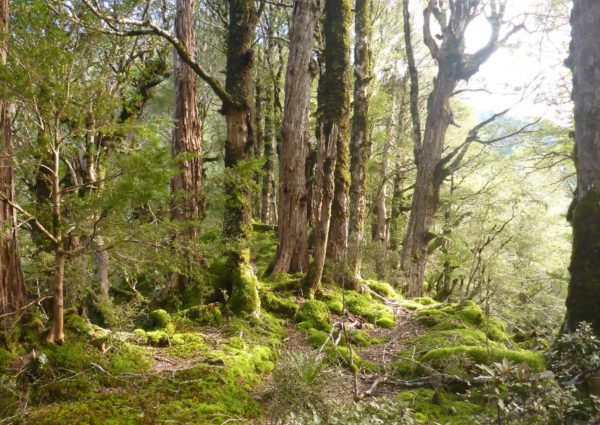
Figure 1: A mature native forest, Ruahine Ranges, New Zealand
Forests as carbon stores and sinks
Forests, being comprised of woody plants at high density, play an important role in regulating carbon dioxide in the atmosphere. Carbon is contained within the living tissue of the plants, and also in dead wood and litter on the forest floor. Through the decay process, dead wood and litter release carbon back to the atmosphere. Forests in which growth rates of living plants exceed decay rates of dead plant material are removing carbon from the atmosphere, and are often referred to as sequestering carbon, or being carbon sinks. This is most significant in regenerating forests. Forests eventually reach “steady state”. At this point, the rate at which carbon is sequestered by growth of living trees is matched by the rate at which it is being released into the atmosphere through decay of dead plant material. At steady state a forest does not sequester carbon, but still serves as a carbon store or reservoir. This steady state lasts until a disturbance event (for example a fire, storm, disease or pest outbreak) causes large scale tree death, after which new growth occurs (shown in Figure 2). In summary, all forests store carbon, but only growing forests also sequester carbon.
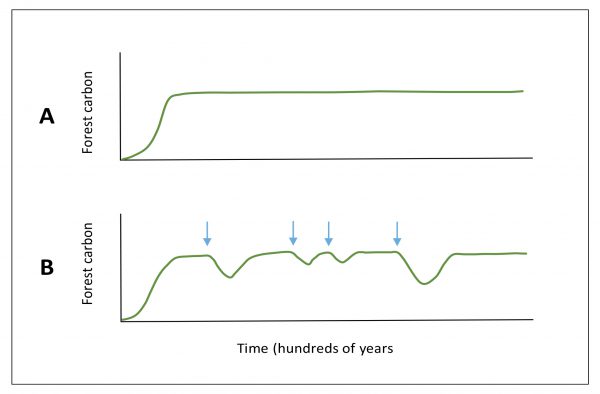
Figure 2: Forest carbon over time. The top figure (A) depicts forest carbon in the absence of disturbance. Carbon stored in the forest increases (i.e. it is a carbon sink) until the amount of carbon being removed from the atmosphere by growth is equal to the amount released through decay (the forest is in steady state). In reality, disturbance occurs and forest carbon fluctuates accordingly, as shown in the lower figure (B). Forest carbon declines following disturbance events (indicated by blue arrows) before increasing again to steady state levels.
Deforestation results in loss of forest carbon reservoirs. Rather than being locked up in the forest, the stored carbon is emitted to the atmosphere. This happens very quickly in the case of forest being burned, and more slowly if timber is harvested and used in wood products or left to decay on site. Huge amounts of deforestation have occurred globally and in New Zealand over the last several hundred years. While increases in atmospheric carbon dioxide concentration are largely the result of fossil fuel use, forest loss has also played a significant role globally (Federici et al 2015). Prior to human arrival in New Zealand, native forests covered 23 million ha, 85% of New Zealand’s land area (Ministry for the Environment, 1997). This has been reduced to just 7.8 million ha, 30% of New Zealand’s land area (Ministry for the Environment, 2018a). Deforestation of New Zealand’s native forest is still occurring, albeit at a much lower rate: between 1990 and 2016 New Zealand lost 66,000 ha of natural (predominantly native) forest (Ministry for the Environment, 2018a).
Studying carbon in New Zealand’s native forests
New Zealand has established a monitoring programme to track carbon storage and sequestration in its native forests at the national scale. This programme has been in operation since 2002 and involves taking forest measurements at around 1200 random sites across the country. The data collected, which include stem diameter, tree height and species, enable calculation of carbon present at the site. Data from all sites are pooled to give average carbon numbers for the whole country. Measurements are taken again at a later date (between 5 and 10 years later), which enables the calculation of change in carbon over time (carbon sequestration rate) [2].
Alongside this, researchers around New Zealand, particularly at the Crown Research Institutes (CRIs) Manaaki Whenua - Landcare Research and Scion, have conducted numerous studies of native forest carbon. This includes studies on biomass, wood density, wood decay rates, and carbon sequestration in a range of forest types including regenerating forest, mature forest, and plantations of native trees. Together, these focussed studies and the nationwide survey, give a picture of carbon dynamics in New Zealand’s native forest.
Carbon stored in New Zealand’s native forests
New Zealand’s remaining native forest provides a significant carbon reservoir. Analysis of the national survey data shows that this forest stores around 850 tonnes of carbon dioxide per hectare (t CO2 / ha) on average at the national scale. Across the 7.8 million ha of this forest that remains in New Zealand, this equates to a store of 6,600 Mt CO2 (Holdaway et al. 2017). Considered alongside global greenhouse gas emissions, this number is relatively large considering New Zealand’s tiny land area. Global greenhouse gas emissions are around 48,000 Mt CO2e annually [3]; the 6600 Mt CO2 stored in New Zealand’s native forests is 13% of this figure [4].
The native forest area consists of both mature forest and regenerating forest (which is recovering from past disturbance, including logging and clearing). Mature forest makes up the majority (6.59 million ha), which has a higher carbon stock per hectare (around 945 t CO2 / ha) than regenerating forest (1.25 million ha at 325 t CO2 / ha) (Holdaway et al. 2014). Podocarp-broadleaved-beech forest has the highest carbon stock per hectare relative to other forest types (Holdaway et al. 2014).
Carbon sequestration rates in New Zealand’s native forests [5]
In any forest, the rate of carbon sequestration changes over time, and this is true for New Zealand’s native forests. Other factors such as forest type and environmental variables also influence sequestration rates, but in a forest that is establishing successfully, a key factor is stage of growth. In regenerating native forest, the rate is slow in the initial stages of development while shrub species take over from grassland. The rate is highest after canopy closure while woody shrubs and small trees (e.g. manuka and kanuka) are growing rapidly. As forest succession progresses, early colonising species are replaced by slower growing tree species. This includes a wider range of broadleaf tree species (e.g. beech species, rata and kamahi), as well as podocarps (e.g. rimu, matai, miro, totara). Sequestration continues until the forest reaches maturity at around 200 – 300 years of age (Carswell et al. 2012). During the first 50 years of growth, a regenerating native forest sequesters, on average, just over 7 t CO2 / ha / yr (Beets et al. 2014) [6]. After 50 years of growth, one hectare of regenerating native forest will have sequestered around 370 t CO2. To put this number in perspective:
- The emissions that result from driving an average small car in New Zealand for one year are approximately 2 t CO2. These emissions could be offset by the growth of just over 0.25 hectares (one quarter of a full sized rugby field) of regenerating native forest for one year [7].
- The emissions from one return flight from Auckland to London for one person are greater (about 3 t CO2) and would require a slightly larger area, closer to 0.5 ha (half a rugby field) [8].
- It would take around 3 years of growth for 1 ha of regenerating native forest to offset one year’s worth of greenhouse gas emissions from an average New Zealand household.
As far as it is possible to tell using current measurements, the mature component of New Zealand’s native forest is currently neither a source nor a sink of carbon at the national scale. The 1.2 million ha of regenerating native forest is growing and sequestering carbon at a rate of between 5.1 and 7.3 t CO2 / ha, depending on its age. This regenerating forest provides a carbon sink of around 6 Mt of CO2 per year (Ministry for the Environment 2018a) [9]. This is equivalent in size to roughly 7% of New Zealand’s gross greenhouse gas emissions in 2016 (Figure 3).
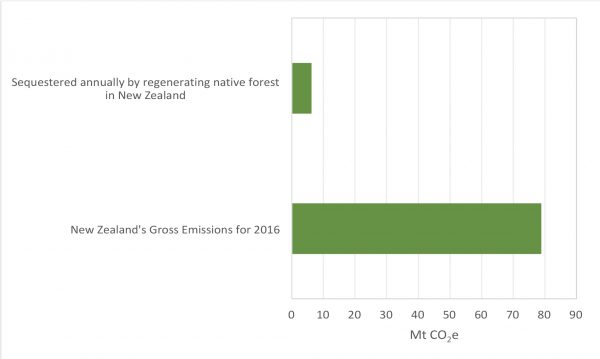
Figure 3: Annual carbon sequestration in New Zealand's existing native forest, compared with New Zealand’s gross emissions for one year (2016). Gross emissions are greenhouse gas emissions associated with agriculture, energy, industrial processes and waste. Data sourced from New Zealand’s Greenhouse Gas Inventory 1990 – 2016 (Ministry for the Environment, 2018a)
The majority of carbon in a forest is stored in the largest trees. In the mature component of New Zealand’s native forest, large trees (>60 cm diameter) comprise only 0.77% of the total number of stems, but contain 40% of the total biomass stocks (Holdaway et al. 2017). Interestingly, the rate at which individual trees increase in biomass (and therefore carbon) also increases with tree size. The oldest and largest trees in a forest can contribute a disproportionate amount of growth in biomass. For example, in USA old-growth forest, it has been found that trees > 100cm in diameter comprised 6% of trees, yet contribute 33% of the annual forest mass growth (Stephenson et al 2014). However patterns for a forest as a whole can be quite different. While rate of biomass increase may be increasing through time for individual trees, the rate of sequestration of the forest as a whole does not necessarily continue to increase. This is because as the forest matures, other population-level factors influence forest biomass and rate of biomass change, including tree mortality (Stephenson et al 2014).
Native tree plantations
Higher rates of carbon sequestration can be achieved for native plantations (compared to regenerating forests), on favourable sites and where appropriate management techniques are used. Native tree species that have been found to have good sequestration rates in plantation conditions are black beech, red beech, and kauri. For example, black beech and kauri can reach over 600 t CO2 / ha in 50 years (compare to 370 t CO2 / ha for regenerating native forest). For further reading on this topic, refer to Kimberley, Bergin & Beets (2014).
How does carbon stored and sequestered in native forest compare to exotic plantation species such as Pinus radiata?
People in the position of establishing, investing financially, or making policy decisions about forests to address climate change are often faced with weighing up the relative benefits of native forest versus exotic planation forests. Each confers different advantages, and the best option depends on the outcome sought.
In New Zealand, native tree species are slower growing than exotic plantation species. The rate of carbon sequestration in a Pinus radiata plantation is much higher than in a regenerating native forest, as illustrated in Figure 4. It would take 200 – 300 years for a newly established native forest to reach what a P. radiata forest can in ca. 30 years (ca. 700-800 t CO2 / ha). In terms of removing carbon dioxide from the atmosphere in the short term, exotic plantations offer a clear advantage.
There is currently 6.5 times more carbon stored in native forests than exotic plantation forests [10] in New Zealand (Ministry for the Environment, 2017). A major contributing factor to this statistic is the larger area of native forest. New Zealand has 7.8 million ha of native forest compared to 2 million ha of plantation forest (Ministry for the Environment, 2018b). However, if the areas were equal in size, native forest would still store more carbon than our exotic plantation forest. This is because on average, more than twice as much carbon is stored per hectare in New Zealand’s mature indigenous forests than in exotic forests planted for wood production (Ministry for the Environment, 2015). The majority of the native forest estate is mature forest and at or near its maximum carbon stock of around 900 t CO2 / ha. In contrast, exotic plantation forest is usually harvested at around 28 years. At this point it has reached 700 - 800 t CO2 / ha, but after replanting it will take another 28 years for forest carbon to reach these levels. Carbon storage in plantation forest intended for harvest is therefore always limited by the harvest cycle. In a permanent native forest, carbon stored will continue to increase for hundreds of years, and remain stored in that forest for the lifetime of the forest. Upon harvest, some carbon from a plantation forest will remain stored in wood products made from harvested timber (for as long as those products last), while some will be lost back to the atmosphere.
In summary, exotic plantation forest will remove more carbon from the atmosphere in the short term. In the long term, permanent native forests will continue to sequester carbon for a longer period of time than a plantation forest, and store more carbon per hectare on average (as carbon stored in a production forest is ultimately limited by harvest).
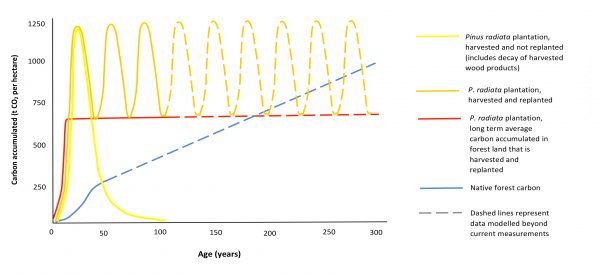
Figure 4: Carbon accumulation in native forest and in plantation Pinus radiata forest in New Zealand. The graph illustrates how P. radiata forest is capable of accumulating carbon much faster than regenerating native forest. However, when the plantation forest is harvested, this carbon is eventually all released back to the atmosphere. If the plantation forest is replanted, carbon will again accumulate, but in the long term, carbon accumulation in plantation forest land is limited by the harvest cycle. In a regenerating native forest (which is not harvested), carbon continues to accumulate and eventually surpasses the long term average of plantation forest land.
The future
The carbon storage and sequestration provided by New Zealand’s existing native forests is sizeable both in a local and a global context. These functions (ecosystem services) will continue to be provided by these forests if they are not deforested or affected by disturbances such as weather, fire or disease outbreaks. Unfortunately, the loss or reduction in area of New Zealand’s native forests would also itself have a significant impact on atmospheric carbon dioxide concentrations as this carbon would be released into the atmosphere. Ironically, one of the predicted effects of climate change in New Zealand is increased risk of disturbance to forests. Climate change will cause increased frequency of extreme weather events including flooding and droughts, as well as an increase in mean temperatures. These factors can cause forest loss both directly and indirectly. In terms of direct effects, flooding can cause landslides resulting in removal of trees, and drought would put stress on lowland forests, also potentially causing partial or total forest loss. Indirectly, warmer temperatures will favour conditions that encourage spread of disease or pests, which could result in dieback or loss of forests or individual tree species (Ministry for the Environment, 2018b). Due to the complex nature of interactions between plants, the abiotic (non-living) environment, and other organisms, exact impacts of climate change on New Zealand’s native forests are difficult to predict. However, evidence shows that changes in climatic conditions can have abrupt and dramatic effects on forests. A well-known example from overseas is the impact of the mountain pine beetle on lodgepole pine forests in British Columbia. The beetle is native, and usually plays a vital and positive part in the functioning of the forest ecosystem. However, following a series of unusually hot summers and mild winters, an unprecedented outbreak of the beetle resulted in large scale forest loss (Green, 2014; Kurz et al 2008).
To substantially increase the amount of carbon stored in native forests in New Zealand, over and above current rates of increase, requires an increase in forest area. Fortunately, there is considerable potential for the establishment of new native forests, and there are multiple environmental benefits associated with doing so. This includes increasing native biodiversity and its gene pool, and in doing this potentially also providing greater resistance to diseases or pests [11], reducing soil erosion, improving water quality and contributing to climate change adaptation.
In terms of biodiversity, native forests contain a diverse range of plants and animals. One of the main threats to native biodiversity in New Zealand is habitat limitation. Re-foresting our landscape with native forests would provide habitat for native plants, fish, birds, bats, and invertebrates. On steep land, native forests provide erosion control. Water quality benefits arise as a result of this erosion control and also intercepting and filtering nutrient run-off from agricultural land. Some of these benefits can also be provided by plantation forests. For example in the case of biodiversity, threatened native bird species such as robins, falcons and kiwi can be found in plantation forests, and plantation forests also provide erosion control and nutrient filtering. But because native forests are (generally) permanent, these benefits are not lost when the forest is harvested [12]. When exotic forests are harvested, the land from which they have been removed can be susceptible to erosion until such a time as forest cover is re-established (generally 5 years) (National Environmental Standards for Plantation Forestry 2017).
To date, financial incentives have not been great enough to encourage significant native afforestation in New Zealand. Around 40,000 ha of land has been converted to native forest in the last 25 years or so. Existing sources of financial support for establishing and maintaining native forests are limited. Government forestry schemes (e.g. the Afforestation Grant Scheme (AGS), the Erosion Control Funding Project (ECFP)) can supplement initial establishment costs. Income can be generated through sustainable timber harvesting (regulated under the Forests Act) [13], participating in the Emissions Trading Scheme (ETS) or Permanent Forest Sink Initiative (PFSI), linking with voluntary carbon offset schemes, and through honey production. Manuka, which is present in the early stages of native forest regeneration, provides flowers that are now a valuable commodity for honey producers. The boom in the manuka honey industry has provided an incentive for the planting of this species. Unfortunately, the interests of honey production do not necessarily align well with those of forest establishment. For honey production, there is interest in planting genotypes that are not necessarily native to the area, maintaining a monocultural crop, or keeping forests at the manuka stage of regeneration, rather than allowing natural succession of a wider range of tree species, including larger tree species which will provide greater carbon sequestration in the long term.
It is possible for native forest to regenerate under unharvested P. radiata plantations, particularly with management interventions such as creation of gaps in the P. radiata canopy, thinning of P. radiata and tree ferns growing underneath them, and under planting with native tree species (Forbes 2015). Use of this approach could provide the advantage of fast carbon sequestration provided by a P. radiata plantation followed by the multiple benefits provided by a mature native forest. However, there is still a lot to learn about the exact conditions under which this would be successful (Wotton & McAlpine 2013). For example, evidence suggests that proximity to an existing native forest as seed source is important, and also that a native forest regenerating in this way may not be exactly the same as one which regenerates on its own (Forbes, 2015). It is also possible for exotic tree species to invade native forest (for example this can be seen in areas near historic forestry planting trials at Hanmer Springs), and wilding pines are a huge problem especially in drier parts of the South Island where seedlings of exotic plantation species can spread very rapidly, outcompeting native plants in the area.
The easiest and cheapest way to establish a native forest is to exclude grazing stock from an area that is close enough to an existing native forest (to provide a seed source), where numbers of wild grazing animals (deer and goats) are low enough that they will not prevent regeneration from occurring. There is significant land area available which meets these criteria [14]. Planting native trees can be used to speed up the regeneration process, or establish forests where forest is unlikely to regenerate naturally because it is a long way from a seed source. The vast majority of land available for conversion to native forest is in private ownership. An important question at this time is: what policy settings will encourage afforestation on this privately owned land? As mentioned above financial incentives are currently not great enough. This topic has been covered in the media recently, particularly in relation to the current government’s Billion Trees programme. The Billion Trees programme has sparked conversation about what sort of trees to plant, including the proportion of the billion trees that should be natives15. There is general support for including a mixture of exotic plantation forest, as well as permanent native forest, as the best approach. This will enable exotic plantation forests to provide urgently needed carbon sequestration, while also providing some long term benefits from native forest.
Summary
Native forests play an important role in storing and sequestering carbon in the New Zealand landscape. They do not sequester carbon as quickly as pine forests, but nonetheless can provide significant carbon stores and sinks, as well as a range of other benefits. There is considerable potential for the establishment of additional native forest in New Zealand, and it has been recognised that there is value in including native forests as part of the current government’s Billion Trees programme. While forests are an important part of tackling climate change, we cannot offset all our emissions using forests. Reductions in emissions of greenhouse gases to the atmosphere will also have to be found in other sectors of the economy.
Further reading
The following two publications provide well written material on carbon storage in native forests, along with some useful graphs:
- Kimberley, Bergin and Beets (2014): Provides an overview of carbon in native forest plantations. It also touches on New Zealand’s native forest inventory: http://www.tanestrees.org.nz/site/assets/files/1069/10_5_carbon_sequestration.pdf
- Parliamentary Commissioner for the Environment’s report “Climate Change and Agriculture: Understanding Our Biological Emissions”. This report provides a summary of New Zealand’s native forest carbon, a comparison with pine, and puts these numbers in the context of the size of NZ’s emissions from agriculture: https://www.pce.parliament.nz/publications/climate-change-and-agriculture- understanding-the-biological-greenhouse-gases
For detailed descriptions of how forest carbon is calculated, refer to:
- New Zealand’s Greenhouse Gas Inventory (Chapter , Land Use Land Use Change and Forestry) http://www.mfe.govt.nz/climate-change/state-of-our-atmosphere-and-climate/new-zealands-greenhouse-gas-inventory
- Peer reviewed publications on carbon stored in New Zealand’s native forests: Holdaway et al (2017) (https://rdcu.be/8JEN) and Beets et al (2014) (https://doi.org/10.3390/f5092230).
References
- To calculate dry mass, plant material is dried in an oven, to remove all moisture, and is then weighed.
- For more information refer to New Zealand’s Greenhouse Gas Inventory, Chapter 6 (Ministry for the Environment 2018a), or http://www.mfe.govt.nz/climate-change/state-of-our-atmosphere-and-climate/measuring-greenhouse-gas-emissions/measuring
- http://archive.stats.govt.nz/browse_for_stats/environment/environmental-reporting-series/environmental-indicators/Home/Atmosphere-and-climate/global-greenhouse-gases.aspx
- But note in this example, we are comparing an annual emission (the amount of greenhouse gases emitted to the atmosphere each year, globally) with a carbon store (which has taken hundreds of years to reach this size). New Zealand’s native forests do not offset 13% of global greenhouse gas emissions.
- Numbers presented here are national averages. Sequestration rates for a particular area of forest will vary based on a number of factors including environmental variables like soil and rainfall. If you are considering establishing a forest carbon sink (for example to enter into a forestry scheme such as the NZ ETS or PFSI), you will need to investigate potential sequestration rates for your block in more detail.
- This number includes carbon contained in live above ground biomass (trunk, branches, stems and leaves), live below ground biomass (roots), dead wood (e.g. dead logs on the forest floor), and litter (small branches and leaf litter on the forest floor). It excludes carbon contained in soil. The same is true for all other numbers given in this paper.
- Car fuel use and driving distance obtained from www.rightcar.co.nz. Assumes 14,000 km travelled per year, fuel use of 6.1L of regular petrol per km, and a conversion factor of 22.961 to convert fuel used to CO2 emissions. CO2 produced are 1.96 t CO2 per year. Regenerating native forest sequesters 7 t CO2 per year, 7/4 = 1.75, which is close to 1.96.
- Flight calculation: from Air New Zealand’s emissions calculator at: https://www.airnewzealand.co.nz/loyaltymodule/form/carbon-emissions-offset. Accessed on 21 January 2019. Emissions from Auckland to London Heathrow return = 2936 kg of CO2 = approximately 3 t CO2.
- In New Zealand’s Greenhouse Gas Inventory this regenerating forest is reported and discussed as two separate components. One is the regenerating component of pre-1990 natural forest. The other is the naturally regenerating component of post-1989 forest. In this document, these numbers are combined for simplicity. The regenerating component of pre-1990 natural forest dominates these numbers because the associated area is much larger. For more information refer to Ministry for the Environment (2018a).
- A graphical illustration of this can be found at the following Ministry for the Environment State of the Environment report page on forest carbon: http://archive.stats.govt.nz/browse_for_stats/environment/environmental-reporting-series/environmental-indicators/Home/Atmosphere-and-climate/forest-carbon-stocks.aspx
- Through increased geographic range and genetic diversity
- Although note that New Zealand native falcons do well in a plantation forest habitat that includes both harvested and unharvested stands: https://www.radionz.co.nz/national/programmes/ourchangingworld/audio/2018675137/nz-falcons-thriving-in-logged-pine-plantations
- Under Part 3A http://www.legislation.govt.nz/act/public/1949/0019/latest/DLM255626.html
- For example, Trotter et al (2005) estimate 1.45 M ha nationallyFor a recent media discussion on the Billion Trees programme, the inclusion of natives, and incentivisation of afforestation on private land, refer to Kathryn Ryan’s interview with MPI on Radio New Zealand on 29 August 2018: https://www.radionz.co.nz/national/programmes/ninetonoon/audio/2018660163/1bn-trees-natives-to-leave-exotics-in-the-shade
Other References
- Beets, P.N.; Kimberley, M.O.; Paul, T.S.H.; Oliver, G.R.; Pearce, S.H.; Buswell, J.M. (2014) The Inventory of Carbon Stocks in New Zealand’s Post-1989 Natural Forest for Reporting under the Kyoto Protocol. Forests 5: 2230-2252
- Carswell, F. E., Burrows, L.E., Hall, G.M.H., Mason, N.W.H & Allen, R.B. (2012). Carbon and plant diversity gain during 200 years of woody succession in lowland New Zealand. New Zealand Journal of Ecology 36 (2) 191 – 202
- Federici, S., Tubiello, F., Salvatore, M., Jacobs, H., Schidhuber, J. (2015) New estimates of CO2 forest emissions and removals: 1990 – 2015. Forest Ecology and Management, special issue: Changes in Global Forest Resources from 1990 to 2015 : doi.org/10.1016/j.foreco.2015.04.022
- Forbes, A. (2015) Non-Harvest Pinus radiata Plantations for Forest Restoration in New Zealand. A thesis submitted in partial fulfilment to the requirements for the Degree of Doctor of Philosophy in Forestry. New Zealand School of Forestry, University of Canterbury
- Holdaway, R.J., Easdale, T.A., Mason, N.W.H., Carswell, F.E. (2014) LUCAS Natural Forest Carbon Analysis. Prepared for the Ministry for the Environment by Landcare Research. Wellington: Ministry for the Environment
- Holdaway, R.J., Easdale, T.A., Carswell, F.E., Richardson, S. J., Peltzer, D. A., Mason, N. W. H., Brandon, A. M. & Coomes, D.A. (2017) Nationally Representative Plot Network Reveals Contrasting Drivers of Net Biomass Change in Secondary and Old-Growth Forests Ecosystems (2017) 20: 944
- Kimberley, M.O., Bergin, D. & Beets, P.N. (2014). Carbon Sequestration by Planted Native Trees and Shrubs. Tanes Tree Trust: Planting and Managing Native Trees. Technical Article No. 10.5
- Kurz, W.A., Dymond, C. C., Stinson, G., Rampley, G. J., E. T. Neilson, E. T., Carroll, A. L. Ebata T. & Safranyik, L. (2008) Mountain pine beetle and forest carbon feedback to climate change. Nature 452: 24. doi:10.1038/nature06777
- Ministry for the Environment (1997) The State of New Zealand’s Environment 1997. Wellington, Ministry for the Environment: https://environment.govt.nz/publications/environmental-reporting/state-%20new-zealand%e2%80%99s-environment-1997-chapter-eight-state-our-2, accessed on 21 January 2019
- Ministry for the Environment (2018a) New Zealand’s Greenhouse Gas Inventory 1990 – 2016. Wellington, Ministry for the Environment
- Ministry for the Environment (2018b) Overview of Climate Change Impacts in New Zealand: http://www.mfe.govt.nz/climate-change/likely-impacts-of-climate-change/overview-of-likely-climate-change-impacts, accessed on 21 January 2019
- Ministry for the Environment & Stats NZ (2017). New Zealand’s Environmental Reporting Series: Our atmosphere and climate 2017. Retrieved from www.mfe.govt.nz and www.stats.govt.nz http://archive.stats.govt.nz/browse_for_stats/environment/environmental-reporting-series/environmental-indicators/Home/Atmosphere-and-climate/forest-carbon-stocks.aspx
- Ministry for Primary Industries (2017) A guide to Carbon Look-up Tables for Forestry in the Emissions Trading Scheme. Wellington, Ministry for Primary Industries
- National Environmental Standards for Plantation Forestry (2017) https://www.mpi.govt.nz/growing-and-harvesting/forestry/national-environmental-standards-for-plantation-forestry
- Trotter, C., Tate, K., Townsend, J., Wilde, H., Lambie, S., Marden, M. & Pinkney, T. (2005). Afforestation / reforestation of New Zealand marginal pasture lands by indigenous shrublands: the potential for Kyoto forest sinks. Ann. For. Sci. 62: 865 – 871
- Wotton, D. M. & McAlpine, KG. (2013) Predicting native plant succession through woody weeds in New Zealand. Department of Conservation report.
This paper was peer reviewed by
Dr. Robert Holdaway, Ecosystems and Global Change, Manaaki Whenua/Landcare Research, Lincoln NZ.
While the reviewer has provided comment on drafts of this article, he does not necessarily endorse it in its final form. The author is solely responsible for any errors and judgements that may exist in the published article.
v1.4 26th February, 2019